All Medical Genetics Summaries content, except where otherwise noted, is licensed under a Creative Commons Attribution 4.0 International (CC BY 4.0) license which permits copying, distribution, and adaptation of the work, provided the original work is properly cited and any changes from the original work are properly indicated. Any altered, transformed, or adapted form of the work may only be distributed under the same or similar license to this one.
NCBI Bookshelf. A service of the National Library of Medicine, National Institutes of Health.
Pratt VM, Scott SA, Pirmohamed M, et al., editors. Medical Genetics Summaries [Internet]. Bethesda (MD): National Center for Biotechnology Information (US); 2012-.
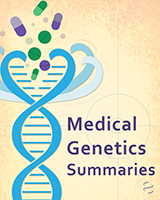
Medical Genetics Summaries [Internet].
Show detailsAtazanavir Therapy and UGT1A1 Genotype
Megan Kane, PhD.
Author Information and AffiliationsCreated: October 17, 2023.
Estimated reading time: 24 minutes
Introduction
Atazanavir is indicated for managing human immunodeficiency virus (HIV) infection as part of a multi-drug regimen (1). While it was once widely recommended as a first-line therapy, it is now primarily suggested as a second-line therapeutic option due to potential adverse effects leading to discontinuation of therapy (2, 3). Atazanavir can cause hyperbilirubinemia (not associated with liver injury) leading to jaundice, which is a common cause of drug discontinuation. Individuals with 2 decreased-function alleles for UGT1A1 are most likely to experience jaundice leading to atazanavir discontinuation, although this can occur despite the individual having a reference UGT1A1 genotype (4). The Clinical Pharmacogenetics Implementation Consortium (CPIC) recommends that when an individual is a known UGT1A1 poor metabolizer, an alternative therapy should be considered particularly when jaundice is of concern to the individual (Table 1) (4). The US Food and Drug Administration (FDA) approved drug label states that certain comedications that depend upon UGT1A1 or the cytochrome P450 family member CYP3A are contraindications for atazanavir therapy due to the potential for elevated plasma concentrations of these comedications (1).
Table 1:
The Clinical Pharmacogenetics Implementation Consortium (CPIC) Recommended Use of Boosted Atazanavir by UGT1A1 Phenotype (2016)
Phenotype | Example UGT1A1 genotypea | Implications for phenotypic measures | Dosing recommendation | Strength of recommendation |
---|---|---|---|---|
Normal metabolizerb |
*1/*1
*1/*36 *36/*36 | Referencec UGT1A1 activity; very low likelihood of bilirubin-related discontinuation of atazanavir | There is no need to avoid prescribing atazanavir based on UGT1A1 genetic test results. Inform the individual that some individuals stop atazanavir because of jaundice (yellow eyes and skin), but that this individual’s genotype makes this unlikely (less than approximately a 1-in-20 chance of stopping atazanavir because of jaundice). | Strong |
Intermediate metabolizer |
*1/*6
*1/*27 *1/*28 *36/*37 | Somewhat decreased UGT1A1 activity; low likelihood of bilirubin-related discontinuation of atazanavir. | Strong | |
Poor metabolizer | *6/*6 *6/*27 *6/*28 *27/*28 *37/*37 | Markedly decreased UGT1A1 activity; high likelihood of bilirubin-related discontinuation of atazanavir | Consider an alternative agent particularly where jaundice would be of concern to the individual. If atazanavir is to be prescribed, there is a high likelihood of developing jaundice that will result in atazanavir discontinuation (at least 20% and as high as 60%). | Strong |
Note: All studies correlating UGT1A1 genotype with atazanavir adverse events have involved ritonavir boosting.
- a
Example genotype (also called a diplotype) data from CPIC Diplotype-Phenotype table, as provided in (5).
- b
Original CPIC guidelines used the term of “extensive metabolizer” which has been substituted for the current, standardized term of “normal metabolizer.”
- c
“Reference” function refers to the UGT1A1 allele to which other alleles are compared.
This table has been adapted from (4).
Drug: Atazanavir
Atazanavir is an antiretroviral protease inhibitor (PI) used to treat HIV infection in adults and pediatric individuals weighing at least 15 kilograms (1, 6, 7). The current standard of care for HIV infection is combination antiretroviral therapy (cART), where multiple classes of antiretroviral medications are taken together (3). Atazanavir is most often prescribed with 2 nucleoside reverse transcriptase inhibitors (NRTIs) and a pharmacokinetic booster––either ritonavir or cobicistat––to slow the metabolism of atazanavir and ensure sufficient plasma levels for therapeutic efficacy (3). Atazanavir-containing ART is recommended by the World Health Organization (WHO) as a second-line drug regimen for individuals for whom a dolutegravir-based first-line therapy has failed (2). However, both the WHO and the U.S. Department of Health and Human Services Panel on Antiretroviral Guidelines for Adults and Adolescents recommend darunavir over atazanavir due to its lower rate of drug discontinuation in response to adverse effects (2, 3). Atazanavir-containing regimens should be avoided in individuals with high viral load (HIV RNA ≥100,000 copies/mL), chronic kidney disease (creatinine clearance <60 mL/min), severe hepatic impairment (Child-Pugh Class C), or a history of clinically significant hypersensitivity (including Stevens-Johnson syndrome, erythema multiforme, or toxic skin eruptions) to components of the medication formulation (1, 3).
Primary metabolism of atazanavir occurs via the cytochrome P450, family 3, subfamily A (CYP3A) enzymes, and both isoenzymes 4 and 5 (CYP3A4/5) (1, 8). Coadministration with ritonavir or cobicistat leads to inhibition of the CYP3A4/5 enzymes and improves pharmacokinetics, decreasing the daily pill burden for the individual (8). Thus, for individuals who can tolerate ritonavir, the recommended daily dose of atazanavir is 300 mg (plus 100 mg of ritonavir); if ritonavir is not well tolerated, the daily dose of atazanavir should be 400 mg (1). Atazanavir is administered orally and should be taken with food to increase overall bioavailability; absorption is rapid, and maximum plasma concentration is reached approximately 2.5 hours after dosing (or up to 5 hours if taken with a high-fat meal) (1). In plasma, atazanavir is 86% bound to proteins, including alpha-1-acid glycoprotein and albumin (1). The WHO recommends avoiding atazanavir with rifampin, a strong CYP3A4 inducer, due to decreased plasma levels of atazanavir (2, 9). Medications that inhibit CYP3A activity can significantly increase plasma atazanavir and ritonavir levels and lead to severe, life-threatening, or fatal events and should be avoided if possible; these medications include amiodarone and indinavir (1, 9). Some medications may be used with altered dosing (see the FDA-approved drug label for full information) (1). Coadministration of ritonavir-boosted atazanavir with the antifungal voriconazole leads to altered pharmacokinetics of voriconazole, especially for individuals with a CYP2C19 genotype associated with poor metabolism of voriconazole; experts recommend using this medication combination only when the benefits outweigh the risks (10).
Atazanavir inhibits the uridine diphosphate-glucuronosyltransferase 1A1 (UGT1A1) enzyme—the only enzyme that conjugates bilirubin for excretion in bile (8, 11). Atazanavir therapy causes an elevation of unconjugated bilirubin in most individuals, though many remain asymptomatic (1). Some individuals can progress to hyperbilirubinemia and jaundice, leading to discontinuation of or non-adherence to cART (4, 12, 13). The increase in unconjugated bilirubin is not associated with other signs of hepatic injury, and any observed elevations of hepatic transaminases should be evaluated for alternative causes (1). Individuals with 2 decreased-function UGT1A1 alleles are at highest risk of bilirubin-related atazanavir discontinuation, although bilirubin elevations can also occur in the absence of this genotype (4).
Other potential side effects associated with atazanavir (with or without ritonavir) include cardiac symptoms, severe skin reactions, liver toxicity in individuals with elevated liver transaminases, development of chronic kidney disease, diabetes mellitus and hyperglycemia, immune reconstitution syndrome, and metabolic abnormalities (1). Cardiac symptoms may include a prolonged duration of the PR interval. The PR interval is a period lasting from the beginning of the P wave (reflecting atrial depolarization) until the beginning of the QRS complex (indicating ventricular depolarization) in an electrocardiogram. The PR interval, sometimes called the PQ interval, is normally between 120 and 200 milliseconds in duration. (14) Reports of prolonged PR intervals associated with atazanavir therapy were not acute but occurred after a few weeks of therapy, either alone or with NRTI (15). Cardiac conduction abnormalities, specifically asymptomatic first-degree atrioventricular (AV) block, were reported in 5.9% of atazanavir-treated individuals (1). In some individuals, the AV block and abnormal ECG rhythm resolved within one week following atazanavir discontinuation, but for others it persisted for one month (15). Individuals with underlying hepatitis B or C infection or preexisting renal disease may require additional monitoring or alternative medications; cases of kidney stones have been reported in individuals during atazanavir therapy and may require temporary interruption of therapy (1). Individuals with hepatic impairment should not take ritonavir to boost atazanavir pharmacokinetics. Thus, individuals with moderate (Child-Pugh class B) hepatic impairment are recommended to be given a daily dose of 300 mg atazanavir without ritonavir boost, while individuals with mild (Class A) hepatic impairment can take the standard 400 mg dose (without ritonavir) (1). Kidney disease, including nephrolithiasis and nephrotoxicity without large calculi, have also been reported in association with atazanavir therapy (16, 17). While PI therapy has been associated with diabetes mellitus and hyperglycemia (1), a pooled meta-analysis found no significant differences in insulin sensitivity during atazanavir therapy (18). Similarly, the reported association of PI therapy leading to increased risk of cardiovascular disease and dyslipidemia is less significant for atazanavir as compared with older PIs (19).
Atazanavir can be used in children, with a recommended daily dose of 200 mg (together with 100 mg ritonavir) in individuals weighing 15-35 kg, and 300 mg daily (with 100 mg ritonavir) for individuals weighing at least 35 kg. Individuals must be 13 years of age and weigh at least 40 kg for unboosted atazanavir therapy, which is dosed at 400 mg per day (1, 20). Increased age is also associated with a higher exposure to atazanavir at standard doses, which may result in a more pronounced decrease in bone mineral density in older adults when compared with darunavir cART (21).
Pregnant women may be prescribed atazanavir and should be given a form of ART to minimize the risk of maternal-fetal HIV transmission (2, 22). The FDA recommends the standard dose of atazanavir for pregnant individuals (300 mg daily with 100 mg ritonavir) (1). Despite the risk of hyperbilirubinemia and jaundice linked to atazanavir therapy, atazanavir was not associated with higher rates of neonatal jaundice (23). Pregnant individuals should not use cobicistat-boosted atazanavir (1, 3) due to reduced exposure and potential loss in efficacy during 2nd and 3rd trimesters (22). One systematic review reported an association of maternal PI therapy with an increased risk of the child being small or very small for gestational age (relative risk 1.24–1.4), though this risk was not significantly different among the multiple PIs studied, including atazanavir (24).
Individuals using hormone-based contraceptive medication may experience drug-drug interactions and altered metabolism when taking boosted-PI based cART. One study reported changes in exposure to etonogestrel (increased) and ethinyl estradiol (decreased) when used concomitantly with atazanavir (25). Similarly, a cobicistat-boosted atazanavir or darunavir regimen was found to increase exposure to drospirenone, leading to an increased risk of hyperkalemia and a subsequent recommendation to avoid atazanavir and cobicistat with drospirenone (24).
Gene: UGT1A1
The UGT enzymes (uridine diphosphate-glucuronosyltransferase) are a superfamily of enzymes that metabolize a wide range of lipophilic molecules, including bilirubin, steroids, toxins, and drugs. These enzymes mediate glucuronidation, a phase II metabolic pathway in which glucuronic acid is conjugated to specific targets to convert them into water-soluble metabolites that can then be eliminated from the body (11).
The UGT genes are polymorphic, and genomic processes such as variant splicing and epigenetic factors likely contribute to their diversity. As a result, the substrates catalyzed by UGT enzymes are particularly variable (26). In humans, the UGT superfamily is made up of 22 enzymes divided into 4 families, of which UGT1A is a member (27). The UGT1A gene locus is a cassette gene located on chromosome 2q37, where common exons 2–5a and 5b are differentially spliced to unique first exons, resulting in the 9 functional UGT1A family members (UGT1A1 and UGT1A3–UGT1A10) (28, 29). The UGT1A1 promoter is regulated differently from other UGT1As and consists of elements sensitive to various substances: xenobiotics (for example, pregnane X receptor (PXR) and constitutive androstane receptor), hydrocarbons (for example, the aryl hydrocarbon receptor), electrophilic nucleophiles and reactive oxygen species (for example, the nuclear factor 2 receptor), endobiotics, and fatty acids (such as the glucocorticoid receptor). The UGT1A1 promoter also contains a critical Thymine-Adenine-Thymine-Adenine (TATA) box that consists of polymorphic tandem repeats, (TA)58TAA. Several CpG islands (DNA regions rich in cytosine-guanine dinucleotide pairs) at the promoter can further alter the affinity and activity of nuclear receptors.
Whereas many UGT enzymes have overlapping glucuronidation substrates, UGT1A1 is the only enzyme that glucuronidates bilirubin, a yellow waste product produced during the catabolism of heme, a constituent of hemoglobin (30). When old or damaged red blood cells are broken down in the spleen, their hemoglobin is broken down to heme, which is then converted to bilirubin. The UGT1A1 enzyme converts this toxic, insoluble form of bilirubin (unconjugated bilirubin) to its non-toxic, soluble form (conjugated bilirubin). Since conjugated bilirubin is water-soluble, it can be dissolved in bile and eliminated with solid waste. If bilirubin is not eliminated and instead builds up to high levels (hyperbilirubinemia), it can cause a yellowish discoloration of the skin and eyes, commonly known as jaundice.
Over 150 genetic variants in the UGT1A1 gene have been reported (11, 30, 31). Of these, the available evidence indicates that 5 polymorphic variants are of clinical importance to UGT1A1 activity (UGT1A1*6, UGT1A1*27, UGT1A1*28, UGT1A1*36, UGT1A1*37); 3 of these variants affect the tandem repeat of the TATA box ((TA)5TAA – UGT1A1*36, (TA)7TAA – UGT1A1*28, (TA)8TAA – UGT1A1*37 (4)). The wild-type allele is called UGT1A1*1, which is associated with normal enzyme activity and the reference TATA box tandem repeat length ((TA)6TAA) (Table 2).
As with all genetic variation, specific alleles or haplotypes can vary in frequency across populations based on genetic ancestry and any history of evolutionary migration or bottleneck. To characterize the range of genetic variation in different populations, studies have used a mix of ethnic, racial, and geographic descriptors to group individuals with assumed common ancestry and shared genetic traits. Those descriptors are used interchangeably below, based on the cited literature; however, the goal is to reflect a shared genetic background arising from common ancestry.
There are multiple genetic variations in the UGT1A1 locus that reduce UGT1A1 enzyme activity and can lead to jaundice in the absence of exogenous substances, such as belinostat. The jaundice may be mild, as seen in Gilbert syndrome, or severe, as observed in Crigler-Najjar syndrome. (12)
The most common variant UGT1A1 allele is UGT1A1*28, which is commonly found in individuals of African descent (“African Americans”; 0.42–0.45 allele frequency, or 17–20% frequency of homozygosity in the population), European descent (“Caucasians”; 0.26–0.31 allele frequency, or 6–9% homozygosity), and in Western and South Asian populations (0.26–0.33 allele frequency, or 6–10% homozygosity). In contrast, it is less common in East and Southeast Asian populations (0.09–0.16 allele frequency, or 0.8–2.5% homozygosity) (32, 33, 34). Within European-and African-descended American populations, the UGT1A1*28 variant is a common cause of Gilbert syndrome (32, 35). The UGT1A1*28 [(TA)7TAA] variant contains an extra thymine-adenine (TA) repeat within the TATA box promoter region (7 TA repeats as opposed to 6 in the wild-type allele) (36). This extra TA repeat reduces the rate of transcription initiation of the UGT1A1 gene, leading to decreased enzyme activity and bilirubin glucuronidation (37). Evidence indicates that one copy of the UGT1A1*28 allele results in an approximately 35% decrease in transcriptional activity, and 2 copies (*28/*28, homozygous) yield an approximate 70% decrease (38, 39).
Another variant allele, UGT1A1*37 [(TA)8TAA], has 8 TA repeats at the TATA box site, and results in reduced promoter activity to levels lower than the UGT1A1*28 allele. In contrast, the UGT1A1*36 [(TA)5TAA] allele only has 5 repeats and is associated with increased promoter activity and a reduced risk of neonatal hyperbilirubinemia (a common and typically benign condition). The UGT1A1*36 and UGT1A1*37 alleles occur almost exclusively in populations of African origin, with estimated allele frequencies across African-descended populations of 0.07 for *36 (TA5) and 0.05 for *37 (TA8) (gnomAD browser version 3.1.2, accessed 27 April 2023) (40). By comparison, the average frequency of these alleles across all populations in gnomAD is 0.01–0.02. The UGT1A1*80 allele exhibits almost complete linkage disequilibrium with both UGT1A1*28 and *37 and can be considered as a surrogate marker for these alleles (4).
Other promoter variants have been reported in the phenobarbital-responsive enhancer module of the UGT1A locus. A thymine (T) to guanine (G) substitution, known as UGT1A1*60, results in decreased transcription and is found more often in individuals with mild hyperbilirubinemia (41). However, other studies indicate no significant difference in total bilirubin concentration between individuals homozygous for UGT1A1*60 versus wild-type homozygotes (42). The UGT1A1*60 allele has been observed more frequently in individuals of African compared to European descent (43). It’s worth noting that the UGT1A1*28 and *60 alleles are reported to be in linkage disequilibrium in multiple ethnic groups (43, 44). This means an individual with a higher number of TA repeats in the promoter (UGT1A1*28) is also likely to have the T to G substitution (UGT1A1*60) in the phenobarbital-responsive enhancer module region. As a result, discerning the individual contribution of these variants to total enzyme activity in vivo can be difficult. The UGT1A1*60 allele has a reported frequency of 0.47 in individuals of European descent and 0.85 in Americans of African descent (43).
Another variant allele, UGT1A1*6, is more prevalent in East Asian populations, with allele frequencies ranging from 0.10–0.30 in Taiwanese, Chinese, Korean, and Japanese populations (34, 38, 45, 46). Conversely, the UGT1A1*6 allele is less common in Southeastern and Southern Asian populations, with frequencies ranging from 0.027–0.12 in Thai, Malay, Indonesian, Vietnamese, and Indian population studies (34). This missense variant results in a glycine to arginine amino acid change at position 71 (p.Arg71Gly), and individuals who are homozygous for this allele have reduced UGT1A1 enzyme activity, which can cause Gilbert syndrome and prolonged neonatal jaundice (47, 48, 49, 50).
The UGT1A1*27 (p.Pro229Gln) variant is in exon 1 and has a minor allele frequency between 0.00011–0.0030 in individuals with Asian ancestry. This allele is associated with Gilbert syndrome, post-irinotecan hyperbilirubinemia, and severe or life-threatening leukopenia or diarrhea during irinotecan therapy (51, 52). The allele is also associated with a significant decrease in UGT1A1 substrate binding and catalytic activity (53).
Table 2:
Relative Enzymatic Activity of UGT1A1 Variants
Allele name | Variant | Relative activity | Potential impact on drug metabolism | CPIC functional statuse |
---|---|---|---|---|
UGT1A1*1 | None (Promoter [TA]6TAA) | 100%a | Normal | Normal function |
UGT1A1*6 | p.Arg71Gly | 70%b | Slower | Decreased function |
UGT1A1*27 | p.Pro229Gln | 50% c | Slower | Decreased function |
UGT1A1*28 | Promoter [TA]7TAA | 65%a | Slower | Decreased function |
UGT1A1*36 | Promoter [TA]5TAA | 130%a | Faster | Increased function |
UGT1A1*37 | Promoter [TA]8TAA | 50%a | Slower | Decreased function |
UGT1A1*60 | c.-3279T>G | 60%d | Slower | Normal functionf |
Other genes of note:
Variation at the NR1I2, ABCB1, and SLCO1B1 loci has been associated with altered atazanavir pharmacokinetics (56, 57, 58, 59). The PXR protein, encoded by NR1I2, controls the expression of several genes involved in drug transport and metabolism, while P-glycoprotein (also known as multi-drug resistant protein and encoded by ABCB1) and OATP1B1 (encoded by SLCO1B1) are membrane transport proteins that may facilitate the movement of atazanavir (8). Multiple studies have associated the C to T variation at rs2472677 in the NR1I2 locus with faster atazanavir clearance (57, 58, 60). One study reported that individuals with at least 2 variants in any of these 3 loci (NG_011856.1:g.24087C>T at rs2472677 in NR1I2, NG_011513.1:g.208920T>C at rs1045642 in ABCB1, and NM_006446.5:c.521T>C at rs4149056 in SLCO1B1) maintained a plasma concentration of atazanavir above 150 ng/mL when the unboosted medication was administered at 200 mg twice daily, rather than 400 mg once daily (56). Variants in these drug transport proteins (or overall reduced expression of multiple transporters) can impact clearance of atazanavir, though no official guidance has been issued to suggest alternative dosing schedules based on these genotypes. Additionally, one small study reported an association of a variant in intron 1 of SORCS2 (rs73208473, NM_001348945.2:c.3645T>G) with decreased plasma concentration and exposure to atazanavir; potentially via miRNA4798 and expression regulation of NR1I2 mRNA (61).
Genetic variations at the CYP3A4 and 5 loci have also been studied for their potential impact on atazanavir pharmacokinetics and clinical outcomes. Studies have reported that CYP3A5 expression is associated with altered metabolism of atazanavir (62) and decreased clearance rates (13). Individuals with 2 no-function alleles of CYP3A5 (such as CYP3A5*3, *6, or *7) have slower oral clearance of unboosted atazanavir compared to those with at least one normal-function allele (8). Similarly, in a study of a Thai population, variation in CYP3A5 was associated with decreased clearance of ritonavir-boosted atazanavir (63). Increased total bilirubin levels were also associated with a variant (rs4253728, NM_001393941.1:c.209-1003G>A) in the PPARA locus, potentially due to the role of peroxisome proliferation-activated receptor alpha as a CYP3A trans-acting factor (64).
Linking Gene Variation with Treatment Response: UGT1A1
Decreased UGT1A1 enzymatic activity, including decreases in activity observed in Gilbert syndrome, is associated with an increased risk of atazanavir discontinuation due to hyperbilirubinemia (65, 66, 67). Individuals who have 2 decreased-function alleles (such as UGT1A1*28 or *37) are at greatest risk for jaundice (4, 68, 69, 70). Significant hyperbilirubinemia (>85 micromol/L) was found in association with a haplotype involving multiple variants at the UGT1A locus affecting 3 members of the UGT1A family (71, 72). Some studies have reported that the correlation between UGT1A1 decreased-function alleles and atazanavir discontinuation is stronger in individuals with lower skin melanin content (Whites), possibly due to jaundice being more noticeable in these individuals (72, 73).
Genetic Testing
The NIH Genetic Testing Registry (GTR) has tests for atazanavir response and UGT1A1 genetic variation. Variants impacting UGT1A1 enzyme activity affect both the coding sequence as well as the promoter region of the UGT1A locus. Genotyping for different lengths of the TA promoter requires a high degree of precision, particularly given the multiple variant alleles reported for that position. It is therefore important to consider the testing methodology when selecting a genetic test or reviewing testing results.
The UGT1A1*28 allele has been reported to be in near complete linkage disequilibrium with the *80 allele; however, the UGT1A1*80 variant itself is not known to influence UGT1A1 expression (74). Instead, UGT1A1*80 has been suggested to serve as a proxy for *28 identification in some genotyping assays (4, 74, 75).
Additionally, UGT1A1 genotyping may reveal variants that are associated with Gilbert syndrome or Crigler-Najjar syndrome type 1 or type 2. Additional information on these conditions is available through MedGen. While more clinical data may be needed on atazanavir metabolism, studies have shown that variants associated with Gilbert syndrome or Crigler-Najjar syndrome type 2 impact the metabolism of bilirubin and multiple exogenous substances (4, 30, 53, 76).
The UGT1A1 Gene Interactions with Medications Used for Additional Indications
Variations in UGT1A1 and its promoter region are associated with risks of adverse reactions for a range of medications.
● Multiple oncology medications including irinotecan, belinostat, nilotinib, and sacituzumab govitecan are metabolized by UGT1A1. Decreased UGT1A1 activity may lead to increased exposure to these medications with a higher risk of adverse reactions; adjusted dosing may be necessary based on UGT1A1 genotype.
- Additional HIV medications, including dolutegravir, are also metabolized by UGT1A1 (77)
- A medication used for acromegalia, pegvisomant, has caused liver injury in individuals positive for UGT1A1*28 (11)
Additional information on gene-drug interactions for UGT1A1 are available from PharmGKB, CPIC and the FDA (search for “UGT1A1”).
Therapeutic Recommendations based on Genotype
This section contains excerpted 1 information on gene-based dosing recommendations. Neither this section nor other parts of this review contain the complete recommendations from the sources.
2023 Statement from the US Food and Drug Administration (FDA)
Contraindications
Atazanavir capsules are contraindicated… when coadministered with drugs that are highly dependent on CYP3A or UGT1A1 for clearance, and for which elevated plasma concentrations of the interacting drugs are associated with serious and/or life-threatening events […]
Drug interactions
Atazanavir is an inhibitor of CYP3A and UGT1A1. Coadministration of atazanavir and drugs primarily metabolized by CYP3A or UGT1A1 may result in increased plasma concentration of the other drug that could increase or prolong its therapeutic and adverse effects. […]
Atazanavir is a CYP3A4 substrate; therefore, drugs that induce CYP3A4 may decrease atazanavir plasma concentrations and reduce atazanavir's therapeutic effect.
Please review the complete therapeutic recommendations that are located here: (1)
2016 Statement from the Clinical Pharmacogenetics Implementation Consortium (CPIC)
For individuals carrying two UGT1A1 decreased function alleles (i.e., UGT1A1*28/*28, UGT1A1*28/*37, UGT1A1*37/*37, or rs887829 T/T), the likelihood of bilirubin-related atazanavir discontinuation is substantial. Before such individuals are prescribed atazanavir (boosted with either ritonavir or cobicistat), all such patients should be advised about the substantial likelihood of developing jaundice. Prescribing atazanavir to such individuals should generally be avoided unless the patient does not consider jaundice to be a concern, or there are other compelling reasons to prescribe atazanavir.
For individuals carrying fewer than two UGT1A1 decreased function alleles (i.e., *1/*28, *1/*37, *36/*28, *36/*37, rs887829 C/C or rs887829 C/T), the likelihood of bilirubin-related atazanavir discontinuation is low. This risk is extremely low for individuals carrying no UGT1A1 decreased function alleles (i.e., UGT1A1*1/*1, UGT1A1*1/*36, UGT1A1*36/*36, or rs887829 C/C). Among patients with extensive metabolizer UGT1A1 phenotypes it may not be necessary to discuss the possibility of jaundice with atazanavir. This decision about whether to discuss possible jaundice should be based on the clinical situation and provider judgment. If advice is offered, such discussion may note that the likelihood of developing jaundice that would require discontinuation of atazanavir is very low.
Please review the complete therapeutic recommendations that are located here: (4)
Nomenclature for Selected UGT1A1 Alleles
Common allele name | Alternative names | HGVS reference sequence | dbSNP reference identifier for allele location | |
---|---|---|---|---|
Coding | Protein | |||
UGT1A1*1 | (TA)6TAA | NM_000463.2:c.-53_-52TA[7] | Not applicable—variant occurs in a non-coding (TATA box promoter) region | rs3064744 |
UGT1A1*6 | 211G>A Gly71Arg | NM_000463.2:c.211G>A (NM_001072.4:c.862-6536G>A) | NP_000454.1:p.Gly71Arg | rs4148323 |
UGT1A1*27 | Pro229Gln | NM_000463.3:c.686C>A | NP_000454.1:p.Pro229Gln | rs35350960 |
UGT1A1*28 | (TA)7TAA | NM_001072.4:c.862-6800AT[8] | Not applicable—variant occurs in a non-coding (TATA box promoter) region | rs3064744 |
UGT1A1*36 | (TA)5TAA | NM_001072.4:c.862-6800AT[6] | Not applicable—variant occurs in a non-coding (TATA box promoter) region | rs3064744 |
UGT1A1*37 | (TA)8TAA | NM_001072.4:c.862-6800AT[9] | Not applicable—variant occurs in a non-coding (TATA box promoter) region | rs3064744 |
UGT1A1*60 | -3263T>G -3279T>G | NM_001072.4:c.862-10021T>G | Not applicable—variant occurs in a non-coding region | rs4124874 |
Pharmacogenetic Allele Nomenclature: International Workgroup Recommendations for Test Result Reporting (78).
UGT Allele nomenclature and definitions are available from (31)
Guidelines for the description and nomenclature of gene variations are available from the Human Genome Variation Society (HGVS). TATA - Thymine-Adenine-Thymine-Adenine
Acknowledgments
The author would like to thank Baralee Punyawudho, PhD, Department of Pharmaceutical Care, Faculty of Pharmacy, Chiang Mai University, Chiang Mai, Thailand; Bronwyn Ramey, PhD, HCLD(ABB), Director of Precision Medicine, LetsGetChecked, New York, NY; and Kandace Schuft, PharmD, Senior Content Management Consultant, Pharmacogenomics, Wolters Kluwer, Hudson, OH, USA for reviewing this summary.
References
- 1.
- ATAZANAVIR SULFATE- atazanavir capsule. Memphis, TN, USA: NorthStar Rx LLC; 2023. Available from: https://dailymed
.nlm .nih.gov/dailymed/drugInfo .cfm?setid=0e152eab-74fb-413f-914d-5b005b58f847 - 2.
- Global HIV, H.a.S.T.I.P., Guidelines Review Committee., Consolidated guidelines on HIV prevention, testing, treatment, service delivery and monitoring: recommendations for a public health approach, WHO, Editor. 2021, World Health Organization, : Geneva. [PubMed: 34370423]
- 3.
- Guidelines for the Use of Antiretroviral Agents in Adults and Adolescents with HIV, Adolescents., P.o.A.G.f.A.a.; [Cited 27 June 2023]. Available from: https:
//clinicalinfo .hiv.gov/en/guidelines /hiv-clinical-guidelines-adult-and-adolescent-arv - 4.
- Gammal, R.S., M.H. Court, C.E. Haidar, O.F. Iwuchukwu, et al., Clinical Pharmacogenetics Implementation Consortium (CPIC) Guideline for UGT1A1 and Atazanavir Prescribing. Clin Pharmacol Ther, 2016. 99(4): p. 363-9. [PMC free article: PMC4785051] [PubMed: 26417955]
- 5.
- UGT1A1 Diplotype-Phenotype Table, CPIC; [Cited Available from: https://cpicpgx
.org/guidelines /guideline-for-atazanavir-and-ugt1a1 /#:~:text=UGT1A1 %20Diplotype%2DPhenotype%20Table - 6.
- Atazanavir: Uses, Interactions, Mechanism of Action, [Cited 27 June 2023]. Available from: https://go
.drugbank.com/drugs/DB01072 - 7.
- Wishart, D.S., Y.D. Feunang, A.C. Guo, E.J. Lo, et al., DrugBank 5.0: a major update to the DrugBank database for 2018. Nucleic Acids Res, 2018. 46(D1): p. D1074-D1082. [PMC free article: PMC5753335] [PubMed: 29126136]
- 8.
- Alvarellos, M., C. Guillemette, R.B. Altman and T.E. Klein, PharmGKB summary: atazanavir pathway, pharmacokinetics/pharmacodynamics. Pharmacogenet Genomics, 2018. 28(5): p. 127-137. [PMC free article: PMC5910198] [PubMed: 29517518]
- 9.
- Cytochrome P450 3A (including 3A4) inhibitors and inducers. Available from: https://www
.uptodate .com/contents/image?imageKey=CARD %2F76992#! - 10.
- Zhu, L., R.J. Bruggemann, J. Uy, A. Colbers, et al., CYP2C19 Genotype-Dependent Pharmacokinetic Drug Interaction Between Voriconazole and Ritonavir-Boosted Atazanavir in Healthy Subjects. J Clin Pharmacol, 2017. 57(2): p. 235-246. [PubMed: 27432796]
- 11.
- Steventon, G., Uridine diphosphate glucuronosyltransferase 1A1. Xenobiotica, 2020. 50(1): p. 64-76. [PubMed: 31092094]
- 12.
- Bhandari, J., P.K. Thada and D. Yadav, Crigler Najjar Syndrome, in StatPearls. 2023: Treasure Island (FL). [PMC free article: PMC562171] [PubMed: 32965842]
- 13.
- Kile, D.A., S. MaWhinney, C.L. Aquilante, J.E. Rower, et al., A population pharmacokinetic-pharmacogenetic analysis of atazanavir. AIDS Res Hum Retroviruses, 2012. 28(10): p. 1227-34. [PubMed: 22394315]
- 14.
- Douedi, S. and H. Douedi, P wave, in StatPearls. 2023: Treasure Island (FL) ineligible companies. Disclosure: Hani Douedi declares no relevant financial relationships with ineligible companies.
- 15.
- Ridjab, D.A., I. Ivan, F. Budiman and D.J. Juliawati, Current evidence for the risk of PR prolongation, QRS widening, QT prolongation, from lopinavir, ritonavir, atazanavir, and saquinavir: A systematic review. Medicine (Baltimore), 2021. 100(31): p. e26787. [PMC free article: PMC8341216] [PubMed: 34397829]
- 16.
- Marinescu, C.I., M. Leyes, M.A. Ribas, M. Penaranda, et al., Relationships between Serum Levels of Atazanavir and Renal Toxicity or Lithiasis. AIDS Res Treat, 2015. 2015: p. 106954. [PMC free article: PMC4439502] [PubMed: 26064679]
- 17.
- Chu, G.J., C. Henderson, L. Evans, K. Howlin, et al., Chronic granulomatous interstitial nephritis and urothelial metaplasia associated with ritonavir-boosted atazanavir: a case study and literature review. Pathology, 2018. 50(5): p. 565-568. [PubMed: 29941201]
- 18.
- Kajogoo, V.D., M. Gorret Atim, D. Amare, M. Geleta, et al., HIV Protease Inhibitors and Insulin Sensitivity: A Systematic Review and Meta-Analysis of Randomized Controlled Trials. Front Pharmacol, 2021. 12: p. 635089. [PMC free article: PMC8591121] [PubMed: 34790115]
- 19.
- Hatleberg, C.I., L. Ryom and C. Sabin, Cardiovascular risks associated with protease inhibitors for the treatment of HIV. Expert Opin Drug Saf, 2021. 20(11): p. 1351-1366. [PubMed: 34047238]
- 20.
- Saint-Lary, L., M.H. Dassi Tchoupa Revegue, J. Jesson, F. Renaud, et al., Effectiveness and Safety of Atazanavir Use for the Treatment of Children and Adolescents Living With HIV: A Systematic Review. Front Pediatr, 2022. 10: p. 913105. [PMC free article: PMC9168429] [PubMed: 35676899]
- 21.
- Jourjy, J., K. Dahl and E. Huesgen, Antiretroviral Treatment Efficacy and Safety in Older HIV-Infected Adults. Pharmacotherapy, 2015. 35(12): p. 1140-51. [PubMed: 26684554]
- 22.
- Salama, E., A.C. Eke, B.M. Best, M. Mirochnick, et al., Pharmacokinetic Enhancement of HIV Antiretroviral Therapy During Pregnancy. J Clin Pharmacol, 2020. 60(12): p. 1537-1550. [PMC free article: PMC8227837] [PubMed: 32798276]
- 23.
- Eley, T., S.P. Huang, F. Conradie, C.D. Zorrilla, et al., Clinical and pharmacogenetic factors affecting neonatal bilirubinemia following atazanavir treatment of mothers during pregnancy. AIDS Res Hum Retroviruses, 2013. 29(10): p. 1287-92. [PMC free article: PMC3785800] [PubMed: 23782005]
- 24.
- Cowdell, I., K. Beck, C. Portwood, H. Sexton, et al., Adverse perinatal outcomes associated with protease inhibitor-based antiretroviral therapy in pregnant women living with HIV: A systematic review and meta-analysis. EClinicalMedicine, 2022. 46: p. 101368. [PMC free article: PMC9061981] [PubMed: 35521067]
- 25.
- Haas, D.W., Y.S. Cramer, C. Godfrey, S.L. Rosenkranz, et al., Pharmacogenetic interactions between antiretroviral drugs and vaginally administered hormonal contraceptives. Pharmacogenet Genomics, 2020. 30(3): p. 45-53. [PMC free article: PMC7398416] [PubMed: 32106141]
- 26.
- Meech, R., D.G. Hu, R.A. McKinnon, S.N. Mubarokah, et al., The UDP-Glycosyltransferase (UGT) Superfamily: New Members, New Functions, and Novel Paradigms. Physiol Rev, 2019. 99(2): p. 1153-1222. [PubMed: 30724669]
- 27.
- Mackenzie, P.I., K.W. Bock, B. Burchell, C. Guillemette, et al., Nomenclature update for the mammalian UDP glycosyltransferase (UGT) gene superfamily. Pharmacogenet Genomics, 2005. 15(10): p. 677-85. [PubMed: 16141793]
- 28.
- van Es, H.H., A. Bout, J. Liu, L. Anderson, et al., Assignment of the human UDP glucuronosyltransferase gene (UGT1A1) to chromosome region 2q37. Cytogenet Cell Genet, 1993. 63(2): p. 114-6. [PubMed: 8467709]
- 29.
- Sissung, T.M., R. Barbier, L.M. Cordes and W.D. Figg, UGT1A1 Polymorphisms and Mutations Affect Anticancer Drug Therapy, in Handbook of Therapeutic Biomarkers in Cancer, S.X. Yang and J.E. Dancey, Editors. 2021: New York.
- 30.
- Strassburg, C.P., Pharmacogenetics of Gilbert's syndrome. Pharmacogenomics, 2008. 9(6): p. 703-15. [PubMed: 18518849]
- 31.
- UGT Official Nomenclature: UGT1A and UGT2B haplotypes and SNPs tables., [Cited March 2018]. Available from: https://www
.pharmacogenomics .pha.ulaval.ca /ugt-alleles-nomenclature/ - 32.
- Beutler, E., T. Gelbart and A. Demina, Racial variability in the UDP-glucuronosyltransferase 1 (UGT1A1) promoter: a balanced polymorphism for regulation of bilirubin metabolism? Proc Natl Acad Sci U S A, 1998. 95(14): p. 8170-4. [PMC free article: PMC20948] [PubMed: 9653159]
- 33.
- Hall, D., G. Ybazeta, G. Destro-Bisol, M.L. Petzl-Erler, et al., Variability at the uridine diphosphate glucuronosyltransferase 1A1 promoter in human populations and primates. Pharmacogenetics, 1999. 9(5): p. 591-9. [PubMed: 10591539]
- 34.
- Huang, M.J., P.L. Chen and C.S. Huang, Bilirubin metabolism and UDP-glucuronosyltransferase 1A1 variants in Asians: Pathogenic implications and therapeutic response. Kaohsiung J Med Sci, 2022. 38(8): p. 729-738. [PubMed: 35942604]
- 35.
- Guillemette, C., Pharmacogenomics of human UDP-glucuronosyltransferase enzymes. Pharmacogenomics J, 2003. 3(3): p. 136-58. [PubMed: 12815363]
- 36.
- ClinVar: UGT1A1*28, [Cited March 20, 2018]. Available from: https://www
.ncbi.nlm .nih.gov/clinvar/variation/12275/ - 37.
- Bosma, P.J., J.R. Chowdhury, C. Bakker, S. Gantla, et al., The genetic basis of the reduced expression of bilirubin UDP-glucuronosyltransferase 1 in Gilbert's syndrome. N Engl J Med, 1995. 333(18): p. 1171-5. [PubMed: 7565971]
- 38.
- Chapter 1 - Principles of Pharmacogenomics: Pharmacokinetic, Pharmacodynamic, and Clinical Implications., Y.W. Francis Lam, L.H.C.; [Cited March 2018]. Available from: https://www
.sciencedirect .com/science/book/9780123919182 - 39.
- Barbarino, J.M., C.E. Haidar, T.E. Klein and R.B. Altman, PharmGKB summary: very important pharmacogene information for UGT1A1. Pharmacogenet Genomics, 2014. 24(3): p. 177-83. [PMC free article: PMC4091838] [PubMed: 24492252]
- 40.
- Karczewski, K.J., L.C. Francioli, G. Tiao, B.B. Cummings, et al., The mutational constraint spectrum quantified from variation in 141,456 humans. Nature, 2020. 581(7809): p. 434-443. [PMC free article: PMC7334197] [PubMed: 32461654]
- 41.
- Sugatani, J., K. Yamakawa, K. Yoshinari, T. Machida, et al., Identification of a defect in the UGT1A1 gene promoter and its association with hyperbilirubinemia. Biochem Biophys Res Commun, 2002. 292(2): p. 492-7. [PubMed: 11906189]
- 42.
- Pasternak, A.L., K.R. Crews, K.E. Caudle, C. Smith, et al., The impact of the UGT1A1*60 allele on bilirubin serum concentrations. Pharmacogenomics, 2017. 18(1): p. 5-16. [PubMed: 27967321]
- 43.
- Innocenti, F., C. Grimsley, S. Das, J. Ramirez, et al., Haplotype structure of the UDP-glucuronosyltransferase 1A1 promoter in different ethnic groups. Pharmacogenetics, 2002. 12(9): p. 725-33. [PubMed: 12464801]
- 44.
- Maruo, Y., C. D'Addario, A. Mori, M. Iwai, et al., Two linked polymorphic mutations (A(TA)7TAA and T-3279G) of UGT1A1 as the principal cause of Gilbert syndrome. Hum Genet, 2004. 115(6): p. 525-6. [PubMed: 15378351]
- 45.
- Akaba, K., T. Kimura, A. Sasaki, S. Tanabe, et al., Neonatal hyperbilirubinemia and a common mutation of the bilirubin uridine diphosphate-glucuronosyltransferase gene in Japanese. J Hum Genet, 1999. 44(1): p. 22-5. [PubMed: 9929972]
- 46.
- Zhang, X., J.F. Yin, J. Zhang, S.J. Kong, et al., UGT1A1*6 polymorphisms are correlated with irinotecan-induced neutropenia: a systematic review and meta-analysis. Cancer Chemother Pharmacol, 2017. 80(1): p. 135-149. [PubMed: 28585035]
- 47.
- Akaba, K., T. Kimura, A. Sasaki, S. Tanabe, et al., Neonatal hyperbilirubinemia and mutation of the bilirubin uridine diphosphate-glucuronosyltransferase gene: a common missense mutation among Japanese, Koreans and Chinese. Biochem Mol Biol Int, 1998. 46(1): p. 21-6. [PubMed: 9784835]
- 48.
- Yamamoto, K., H. Sato, Y. Fujiyama, Y. Doida, et al., Contribution of two missense mutations (G71R and Y486D) of the bilirubin UDP glycosyltransferase (UGT1A1) gene to phenotypes of Gilbert's syndrome and Crigler-Najjar syndrome type II. Biochim Biophys Acta, 1998. 1406(3): p. 267-73. [PubMed: 9630669]
- 49.
- Maruo, Y., K. Nishizawa, H. Sato, Y. Doida, et al., Association of neonatal hyperbilirubinemia with bilirubin UDP-glucuronosyltransferase polymorphism. Pediatrics, 1999. 103(6 Pt 1): p. 1224-7. [PubMed: 10353933]
- 50.
- Boyd, M.A., P. Srasuebkul, K. Ruxrungtham, P.I. Mackenzie, et al., Relationship between hyperbilirubinaemia and UDP-glucuronosyltransferase 1A1 (UGT1A1) polymorphism in adult HIV-infected Thai patients treated with indinavir. Pharmacogenet Genomics, 2006. 16(5): p. 321-9. [PubMed: 16609363]
- 51.
- Koiwai, O., M. Nishizawa, K. Hasada, S. Aono, et al., Gilbert's syndrome is caused by a heterozygous missense mutation in the gene for bilirubin UDP-glucuronosyltransferase. Hum Mol Genet, 1995. 4(7): p. 1183-6. [PubMed: 8528206]
- 52.
- Ando, Y., H. Saka, M. Ando, T. Sawa, et al., Polymorphisms of UDP-glucuronosyltransferase gene and irinotecan toxicity: a pharmacogenetic analysis. Cancer Res, 2000. 60(24): p. 6921-6. [PubMed: 11156391]
- 53.
- Udomuksorn, W., D.J. Elliot, B.C. Lewis, P.I. Mackenzie, et al., Influence of mutations associated with Gilbert and Crigler-Najjar type II syndromes on the glucuronidation kinetics of bilirubin and other UDP-glucuronosyltransferase 1A substrates. Pharmacogenet Genomics, 2007. 17(12): p. 1017-29. [PubMed: 18004206]
- 54.
- UGT1A1 Allele Functionality Table, CPIC; [Cited 22 June 2023]. Available from: https://files
.cpicpgx .org/data/report/current /allele_function_reference /UGT1A1_allele _functionality_reference.xlsx - 55.
- CPIC. CPIC Guideline for Atazanavir and UGT1A1, March 2017 Update. 2017 13 Feb 2023; Available from: https://cpicpgx
.org/guidelines /guideline-for-atazanavir-and-ugt1a1/. - 56.
- Bonora, S., S. Rusconi, A. Calcagno, M. Bracchi, et al., Successful pharmacogenetics-based optimization of unboosted atazanavir plasma exposure in HIV-positive patients: a randomized, controlled, pilot study (the REYAGEN study). J Antimicrob Chemother, 2015. 70(11): p. 3096-9. [PubMed: 26174719]
- 57.
- Siccardi, M., A. D'Avolio, L. Baietto, S. Gibbons, et al., Association of a single-nucleotide polymorphism in the pregnane X receptor (PXR 63396C-->T) with reduced concentrations of unboosted atazanavir. Clin Infect Dis, 2008. 47(9): p. 1222-5. [PMC free article: PMC3672984] [PubMed: 18831695]
- 58.
- Schipani, A., M. Siccardi, A. D'Avolio, L. Baietto, et al., Population pharmacokinetic modeling of the association between 63396C->T pregnane X receptor polymorphism and unboosted atazanavir clearance. Antimicrob Agents Chemother, 2010. 54(12): p. 5242-50. [PMC free article: PMC2981241] [PubMed: 20921307]
- 59.
- Mbatchi, L.C., J.P. Brouillet and A. Evrard, Genetic variations of the xenoreceptors NR1I2 and NR1I3 and their effect on drug disposition and response variability. Pharmacogenomics, 2018. 19(1): p. 61-77. [PubMed: 29199543]
- 60.
- Svard, J., J.P. Spiers, F. Mulcahy and M. Hennessy, Nuclear receptor-mediated induction of CYP450 by antiretrovirals: functional consequences of NR1I2 (PXR) polymorphisms and differential prevalence in whites and sub-Saharan Africans. J Acquir Immune Defic Syndr, 2010. 55(5): p. 536-49. [PubMed: 20861742]
- 61.
- Tamraz, B., Y. Huang, A.L. French, S. Kassaye, et al., Association of Pharmacogenetic Markers With Atazanavir Exposure in HIV-Infected Women. Clin Pharmacol Ther, 2020. 107(2): p. 315-318. [PMC free article: PMC10810687] [PubMed: 31562781]
- 62.
- Castillo-Mancilla, J.R., C.L. Aquilante, M.F. Wempe, L.M. Smeaton, et al., Pharmacogenetics of unboosted atazanavir in HIV-infected individuals in resource-limited settings: a sub-study of the AIDS Clinical Trials Group (ACTG) PEARLS study (NWCS 342). J Antimicrob Chemother, 2016. 71(6): p. 1609-18. [PMC free article: PMC4867099] [PubMed: 26892777]
- 63.
- Singkham, N., A. Avihingsanon, R.C. Brundage, A.K. Birnbaum, et al., Pharmacogenetics-based population pharmacokinetic analysis for dose optimization of ritonavir-boosted atazanavir in Thai adult HIV-infected patients. Expert Rev Clin Pharmacol, 2022. 15(1): p. 99-108. [PubMed: 34727835]
- 64.
- Falvella, F.S., E. Ricci, S. Cheli, C. Resnati, et al., Pharmacogenetics-based optimisation of atazanavir treatment: potential role of new genetic predictors. Drug Metab Pers Ther, 2017. 32(2): p. 115-117. [PubMed: 28599374]
- 65.
- Rotger, M., P. Taffe, G. Bleiber, H.F. Gunthard, et al., Gilbert syndrome and the development of antiretroviral therapy-associated hyperbilirubinemia. J Infect Dis, 2005. 192(8): p. 1381-6. [PubMed: 16170755]
- 66.
- Lubomirov, R., S. Colombo, J. di Iulio, B. Ledergerber, et al., Association of pharmacogenetic markers with premature discontinuation of first-line anti-HIV therapy: an observational cohort study. J Infect Dis, 2011. 203(2): p. 246-57. [PMC free article: PMC3071070] [PubMed: 21288825]
- 67.
- Ribaudo, H.J., E.S. Daar, C. Tierney, G.D. Morse, et al., Impact of UGT1A1 Gilbert variant on discontinuation of ritonavir-boosted atazanavir in AIDS Clinical Trials Group Study A5202. J Infect Dis, 2013. 207(3): p. 420-5. [PMC free article: PMC3537445] [PubMed: 23148286]
- 68.
- Rodriguez-Novoa, S., L. Martin-Carbonero, P. Barreiro, G. Gonzalez-Pardo, et al., Genetic factors influencing atazanavir plasma concentrations and the risk of severe hyperbilirubinemia. AIDS, 2007. 21(1): p. 41-6. [PubMed: 17148966]
- 69.
- Poblete, D., F. Bernal, G. Llull, S. Archiles, et al., Pharmacogenetic Associations Between Atazanavir/UGT1A1*28 and Efavirenz/rs3745274 (CYP2B6) Account for Specific Adverse Reactions in Chilean Patients Undergoing Antiretroviral Therapy. Front Pharmacol, 2021. 12: p. 660965. [PMC free article: PMC8170096] [PubMed: 34093191]
- 70.
- Panagopoulos, P., E. Maltezos, A. Hatzakis and D. Paraskevis, Hyperbilirubinemia in atazanavir treated HIV-infected patients: the impact of the UGT1A1*28 allele. Pharmgenomics Pers Med, 2017. 10: p. 205-208. [PMC free article: PMC5488765] [PubMed: 28790862]
- 71.
- Lankisch, T.O., U. Moebius, M. Wehmeier, G. Behrens, et al., Gilbert's disease and atazanavir: from phenotype to UDP-glucuronosyltransferase haplotype. Hepatology, 2006. 44(5): p. 1324-32. [PubMed: 17058217]
- 72.
- Vardhanabhuti, S., H.J. Ribaudo, R.J. Landovitz, I. Ofotokun, et al., Screening for UGT1A1 Genotype in Study A5257 Would Have Markedly Reduced Premature Discontinuation of Atazanavir for Hyperbilirubinemia. Open Forum Infect Dis, 2015. 2(3): p. ofv085. [PMC free article: PMC4498287] [PubMed: 26180834]
- 73.
- Leger, P., S. Chirwa, J.N. Nwogu, M. Turner, et al., Race/ethnicity difference in the pharmacogenetics of bilirubin-related atazanavir discontinuation. Pharmacogenet Genomics, 2018. 28(1): p. 1-6. [PMC free article: PMC5726942] [PubMed: 29117017]
- 74.
- Bravo-Gomez, A., S. Salvador-Martin, P. Zapata-Cobo, M. Sanjurjo-Saez, et al., Genotyping of UGT1A1*80 as an Alternative to UGT1A1*28 Genotyping in Spain. Pharmaceutics, 2022. 14(10). [PMC free article: PMC9610287] [PubMed: 36297516]
- 75.
- Reizine, N.M., K. Danahey, T.M. Truong, D. George, et al., Clinically actionable genotypes for anticancer prescribing among >1500 patients with pharmacogenomic testing. Cancer, 2022. 128(8): p. 1649-1657. [PMC free article: PMC9153953] [PubMed: 35090043]
- 76.
- Argevani, L., C. Hughes and M.J. Schuh, Dosage Adjustment of Irinotecan in Patients with UGT1A1 Polymorphisms: A Review of Current Literature. Innov Pharm, 2020. 11(3). [PMC free article: PMC8075136] [PubMed: 34007623]
- 77.
- Chen, S., P. St Jean, J. Borland, I. Song, et al., Evaluation of the effect of UGT1A1 polymorphisms on dolutegravir pharmacokinetics. Pharmacogenomics, 2014. 15(1): p. 9-16. [PubMed: 24329186]
- 78.
- Kalman, L.V., J. Agundez, M.L. Appell, J.L. Black, et al., Pharmacogenetic allele nomenclature: International workgroup recommendations for test result reporting. Clin Pharmacol Ther, 2016. 99(2): p. 172-85. [PMC free article: PMC4724253] [PubMed: 26479518]
Footnotes
- 1
The FDA labels specific drug formulations. In this excerpt, we have substituted the generic names for any specific drug labels. The FDA may not have labeled all formulations containing the generic drug. Where necessary, certain terms, genes and genetic variants may be corrected in accordance with nomenclature standards. We have provided the full name of abbreviations, shown in square brackets, where necessary.
- PubReader
- Print View
- Cite this PageKane M. Atazanavir Therapy and UGT1A1 Genotype. 2023 Oct 17. In: Pratt VM, Scott SA, Pirmohamed M, et al., editors. Medical Genetics Summaries [Internet]. Bethesda (MD): National Center for Biotechnology Information (US); 2012-.
- PDF version of this page (511K)
- PDF version of this title (10M)
- Review Clinical Pharmacogenetics Implementation Consortium (CPIC) Guideline for UGT1A1 and Atazanavir Prescribing.[Clin Pharmacol Ther. 2016]Review Clinical Pharmacogenetics Implementation Consortium (CPIC) Guideline for UGT1A1 and Atazanavir Prescribing.Gammal RS, Court MH, Haidar CE, Iwuchukwu OF, Gaur AH, Alvarellos M, Guillemette C, Lennox JL, Whirl-Carrillo M, Brummel SS, et al. Clin Pharmacol Ther. 2016 Apr; 99(4):363-9. Epub 2015 Nov 9.
- Review Effect of the UGT1A1*28 allele on unconjugated hyperbilirubinemia in HIV-positive patients receiving Atazanavir: a systematic review.[Ann Pharmacother. 2013]Review Effect of the UGT1A1*28 allele on unconjugated hyperbilirubinemia in HIV-positive patients receiving Atazanavir: a systematic review.Culley CL, Kiang TK, Gilchrist SE, Ensom MH. Ann Pharmacother. 2013 Apr; 47(4):561-72. Epub 2013 Apr 2.
- Screening for UGT1A1 Genotype in Study A5257 Would Have Markedly Reduced Premature Discontinuation of Atazanavir for Hyperbilirubinemia.[Open Forum Infect Dis. 2015]Screening for UGT1A1 Genotype in Study A5257 Would Have Markedly Reduced Premature Discontinuation of Atazanavir for Hyperbilirubinemia.Vardhanabhuti S, Ribaudo HJ, Landovitz RJ, Ofotokun I, Lennox JL, Currier JS, Olson LM, Haas DW. Open Forum Infect Dis. 2015 Sep; 2(3):ofv085. Epub 2015 Jul 1.
- Impact of UGT1A1 Gilbert variant on discontinuation of ritonavir-boosted atazanavir in AIDS Clinical Trials Group Study A5202.[J Infect Dis. 2013]Impact of UGT1A1 Gilbert variant on discontinuation of ritonavir-boosted atazanavir in AIDS Clinical Trials Group Study A5202.Ribaudo HJ, Daar ES, Tierney C, Morse GD, Mollan K, Sax PE, Fischl MA, Collier AC, Haas DW, DS Clinical Trials Group. J Infect Dis. 2013 Feb 1; 207(3):420-5. Epub 2012 Nov 12.
- Association between the UGT1A1*28 allele and hyperbilirubinemia in HIV-positive patients receiving atazanavir: a meta-analysis.[Biosci Rep. 2019]Association between the UGT1A1*28 allele and hyperbilirubinemia in HIV-positive patients receiving atazanavir: a meta-analysis.Du P, Wang A, Ma Y, Li X. Biosci Rep. 2019 May 31; 39(5). Epub 2019 May 2.
- Atazanavir Therapy and UGT1A1 Genotype - Medical Genetics SummariesAtazanavir Therapy and UGT1A1 Genotype - Medical Genetics Summaries
Your browsing activity is empty.
Activity recording is turned off.
See more...